
The Limits of Energy
Energy is a key driving force of society, embedded everywhere in our lives from iPhones to toaster ovens. However, with a worldwide power demand of 17 terawatts, or 17 trillion watts, per year, our world seems to be reaching the limits of our current energy sources. Moreover, conventional fossil fuels sources, such as oil and coal, cause numerous environmental and health concerns. Thus, much research is focused on developing alternative and renewable energy sources. One of the most promising of these renewable sources is the sun, which provides over 100,000 terawatts of energy to the Earth every year. In nature, plants have taken advantage of this abundance through photosynthesis, a chemical process that converts light energy into the energy of chemical bonds. Today, scientists are trying to mimic this process through “bio-inspired artificial photosynthesis.” Dr. Gary Brudvig, Benjamin Silliman Professor of Chemistry, is one of the scientists investigating this emerging field as part of the collaborative Yale Solar Group, along with Professors Robert Crabtree, Charles Schmuttenmaer, and Victor Batista of the Yale Chemistry Department. A key goal of this investigation is to produce solar fuels, or fuels generated from light sources, as an alternative to the fuels being used today.

Ever since Brudvig came to Yale, his group has studied how nature conducts photosynthesis, arriving at the question, “How does nature oxidize water in the process of solar energy conversion?” Biologically, these reactions occur in Photosystem II, a part of the light-dependent reactions in plant photosynthesis where water oxidation reactions split water molecules into hydrogen ions, oxygen gas, and electrons. In nature, the oxygen gas produced is critical for human life. With artificial methods, the products of this process, particularly the hydrogen ions and electrons, can be further used to generate solar fuels. Therefore, human-designed water oxidation reactions could provide a powerful tool for energy generation and storage.
The water oxidation reaction, however, is both an energy-intensive and mechanistically complex reaction; numerous bonds must be broken and re-formed before the final hydrogen, oxygen, and electron products are created. As a result, this process requires a suitable catalyst for practical application. Even with a catalyst, substantial energy input from light is required for the reaction to occur. Recent research from Brudvig and the Yale Solar Group addresses these dual challenges of catalyst design and light-driven water oxidation.
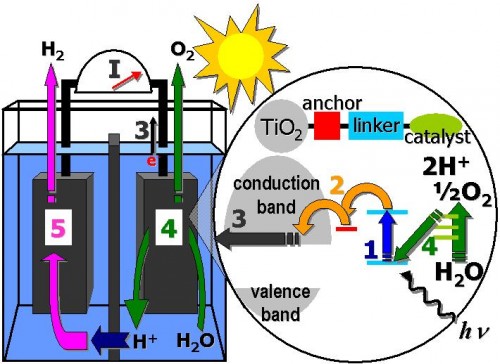
Photoelectrochemical Cells: Nature-Inspired Breakthroughs
The Yale Solar Group currently seeks to use this water oxidation process for energy applications in conjunction with photoelectrochemical cells. Brudvig summarizes this goal succinctly: “The overall process would be the conversion of light into the chemical products of water oxidation.” While variations on photoelectrochemical cells exist, the Yale Solar Group focuses on a design which, in its basic form, consists of a separate photoanode and a cathode immersed in water. The photoanode generates electrons from interactions with light. The cathode, in contrast, accepts these free electrons. The water separates the anode and cathode while serving as the source for water oxidation.
Advances made by the Yale Solar Group working with photoelectrochemical cells have improved the outlook of light-driven water oxidation for energy applications. One particular development is their design of a unique and promising photoanode. The Yale Solar Group took titanium dioxide nanoparticles and attached both zinc porphyrin and iridium catalyst to the particles to create a photoanode especially suited to their photoelectrochemical cell. Titanium dioxide does not absorb visible light itself, so scientists must make modifications to this chemical in order for light interactions to occur. Thus, members of the Yale Solar Group attached zinc porphyrin, a molecule related to light-absorbing structures like chlorophyll, to the titanium dioxide nanoparticle. The choice of zinc porphyrin follows its high coloration, which makes it suitable for greater degrees of visible light absorption, and its well-known stability, which allows for the increased durability of the system. Alongside the zinc porphyrin, an iridium-based catalyst also bonds to the titanium dioxide nanoparticles. The coupling mechanism of these two attachments is such that the absorption of visible light induces the catalysis of water oxidation in the photoelectrochemical cell.
While the Brudvig Lab is currently in the process of incorporating this photoanode into a working photoelectrochemical cell, tests so far have pointed towards a bright future for this material. Brudvig’s lab has so far confirmed the structures and chemical behaviors of its designed photoanode through tests like nuclear magnetic resonance (NMR) and X-rays. Experimentally, Brudvig demonstrated the effectiveness of this photoanode through various means. One experiment is photocurrent measurement on the photoanode; this test essentially inputs a light source onto the photoanode and measures the response in the form of electrical current between the designed photoanode and a standard cathode, in this case platinum. The measured current indicates the effectiveness of electron transfer between the anode and cathode in response to light, and a higher measured current is desired.
Photocurrent measurements on Brudvig’s specially designed photoanodes showed both a relatively large and well-sustained current. However, Brudvig’s group found that when their photoanodes lacked either the zinc porphyrin molecule or the iridium catalyst, the resulting current was much weaker, indicating that both zinc porphyrin and iridium catalyst components were crucial to photoanode performance. It is the hope of the Yale Solar Group that once the anode and cathode are immersed in water to complete a full photoelectrochemical cell, the production of oxygen in the product will indicate successful and sustained light-driven water oxidation.
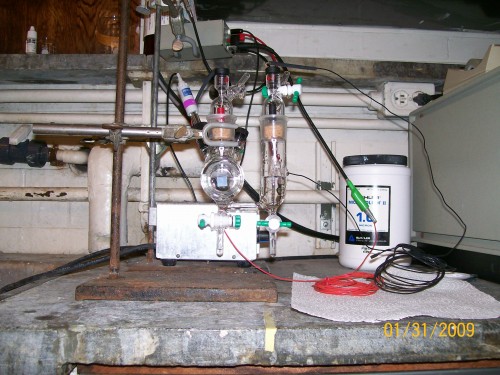
The Solar Energy Challenge
Despite such solar cells’ promise of renewable energy, several key obstacles remain. “These parts all work together,” describes Brudvig, and as such, his lab currently focuses on using multiple approaches to overcome these obstacles. First, photoelectrochemical cells typically have efficiencies of approximately 10 percent, a figure that must increase in order for solar cells to even be considered for practical use. A major source of this inefficiency is the loss of charge separation in the photoelectrochemical cell; division of electron and proton charges between the anode and cathode drives the chemical process within the cell. Recently, Brudvig and his collaborators have been studying molecular rectifiers, a class of molecules which promotes one-way electron transfer, to address this inefficiency, and they are eager to investigate improvements of this design.
Another area of current interest is the development of new catalysts for the water oxidation process. The iridium-based catalyst currently used in many photochemical cell designs is effective, yet quite expensive and rare. Nature, in contrast, uses a manganese product for these water oxidation reactions. As a result, Brudvig is eager to use the insights developed from the studies of iridium-based catalysts and biological manganese compounds to develop catalysts based on more abundant materials.
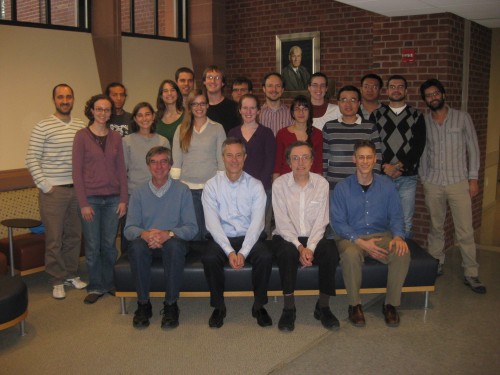
Within the Yale Solar Group, Brudvig looks forward to addressing the key challenges to artificial photosynthesis. At Yale, Brudvig envisions great progress for energy research. He is particularly excited about the Energy Sciences Institute, recently launched on Yale’s West Campus, as it grows in size and scope through the recruitment of new faculty and expansion of current research.
Clearly, many challenges remain before the desired breakthrough in solar energy technology can occur. “We’re just getting started,” states Brudvig. Yet, given the research activity in Brudvig’s lab, at Yale, and abroad, the future of alternative energy remains promising.
About the Author
Jonathan Hwang is a senior chemical engineering major in Saybrook College. He is interested in energy-related topics and works in the Transformative Materials and Devices Lab at Yale under Dr. Andre Taylor.
Acknowledgements
The author would like to thank Professor Gary Brudvig for taking the time to describe artificial photosynthesis research.