Three and a half million years before a thatch-work of roads blanketed the city and decorative trees arose to dot the sidewalks, New Haven belonged to a greener, wetter, and warmer Earth. Absent were today’s neatly spaced regiments of maples and mottled London Planes; vast, uninhibited swaths of green reigned. In those days—the mid-Pliocene Epoch, to be exact—the walk up Prospect Street to climate scientist Nadine Unger’s Yale office would have made a noticeably different trek. Even the air—now with its faint taste of smog—would have contained a different cocktail of ozone, aerosols, and other chemicals than that which we currently breathe.
Dr. Unger, a professor and climate scientist, is especially qualified to explain those differences. Using advanced computer modeling techniques to recreate past climates—and the mid-Pliocene climate in particular—she studies the historical relationship between topography, atmospheric chemistry and Earth’s global average surface air temperature. Her research has helped explain that the atmosphere is influenced by vegetal land-cover, and has also deepened scientific understanding of how various environmental factors influence global warming.
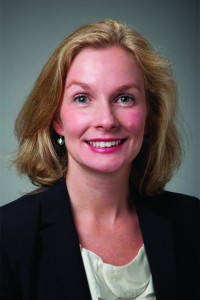
The mid-Pliocene Epoch is especially relevant to Unger’s work because even as the last time in history that the Earth was “significantly warmer” than it is now, the period matched the modern climate state almost exactly in terms of two important variables: atmospheric carbon dioxide (CO2) and arrangement of the continents. Since both of these shared traits are external “forcings,” or factors that are known to control the global climate, scientists have treated the mid-Pliocene as a predictor of Earth’s warming climate. Unger agrees that study of the middle Pliocene warm period is useful for understanding the contemporary issue of Earth system climate sensitivity, but she does not over-analogize the two periods. Instead, her studies of climate and atmospheric chemistry take advantage of the similarities in CO2 and global geography to isolate a factor that has changed drastically in the last few millennia: Earth’s green cover.
Searching for a Greener Earth
Compared to modern times, mid-Pliocene Earth was impressively verdant. The landscape was dominated by lush tropical rainforests and savannas (22 percent and 17 percent vegetated landcover, respectively), with generous expanses of grassland and deciduous forests. It is easy to imagine Australopithecus afarensis, the tree-climbing but still human-like hominin of mid-Pliocene Eastern Africa, surveying that vast, green landscape—scoping out the fruit, seeds, roots, and insects that made up her diet; searching for small lizards to augment her meal; perhaps occasionally pausing halfway up a bole to look out across what must have been a beautiful view.
If suddenly transported through time and space to stand alongside Au. afarensis, Dr. Unger would gaze on the Pliocene topography with a differently expert eye, cataloguing not sources of sustenance, but atmospheric chemicals that influence the climate.
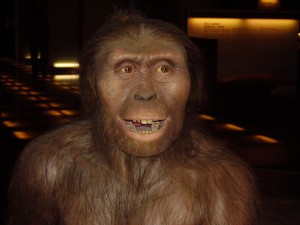
Until recently, little was understood about how changes in land cover impact the atmosphere’s chemical makeup. Unger and her research team, including postdoctoral associate Xu Yue, have set out to change that. In a recent paper entitled “Strong Chemistry-Climate Feedbacks in the Pliocene,” the scientists present evidence that the atmospheric chemicals released by vegetation—either during life or in the process of wildfire-burning—play key roles in climate change.
When the Trees Sigh
To a layperson, the exchange of gases between plants and the atmosphere might seem simple. We have all learned that plants “inhale” carbon dioxide for photosynthesis, and that they breathe out a stream of oxygen. However, according to Unger, the lung analogy fails to capture the more complex reality of trees’ and plants’ relationship with the atmosphere. Terrestrial ecosystems naturally emit not only CO2, but also significant volumes of biogenic volatile organic compounds (BVOCs). Along with methane emitted by wetlands, these chemicals combine with reactive nitrogen compounds from soils, lightning, and wildfire to give rise to ozone (O3). This molecule, one of the most infamous, rapidly reactive greenhouse gases, traps the sun’s energy and has a warming effect on the atmosphere.
In addition to ozone, vegetative land cover also introduces aerosols and their precursors. Aerosols, which are particles suspended in the atmosphere, have complex effects on the climate. Some—such as the primary organic matter, nitrates, and sulfates whose precursors arise from wildfires, soils and volcanoes—can cool the Earth by scattering solar radiation away from the planet. But some of these gases are also precursors for warming O3; and black carbon, the soot released as vegetation burns, absorbs light and causes the atmosphere’s temperature to increase. Wildfires are fueled by organic matter, so the more vegetation covers the land, the greater the effect of atmospheric agents released during wildfires. “Land cover change affects the chemical composition of the atmosphere,” said Unger, “which in turn affects climate change.”
Unger’s Evidence
The overall design of Unger’s paper was to compare the mid-Pliocene and the 1750s preindustrial period, epochs whose climate conditions differed in terms of temperature and vegetation but were otherwise similar. Implementing their own terrestrial biosphere model (the Yale Terrestrial Biosphere Model, which includes climate-sensitive emissions from trees, plants and soils) into NASA’s Model-E2 global Earth System Model, Unger and her team simulated the terrestrial ecosystem emissions and atmospheric chemical composition for each time period. The simulations were highly dynamic, reflecting feedbacks from physical changes in climate on atmospheric reactivity.
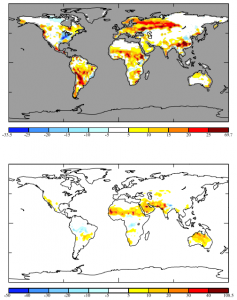
(Bottom panel) Another graphic from Unger’s paper, this map represents the change in soot emission from wildfires between the Mid-Pliocene and preindustrial periods (1×10-12 kg m-2s-1). The color-coding functions the same way as in the isoprene emission map. (Image courtesy of Nadine Unger.)
Upon completion of the simulations, Unger and her team compared the mid-Pliocene data to the preindustrial model. This comparison allowed them to quantify the individual effects of ozone and five aerosols—black carbon, primary organic matter, BSOA, sulfate, and nitrate—on the climate. They also ran a mid-Pliocene simulation that was artificially subjected to the preindustrial vegetation cover conditions. This extra simulation was essential for assessing how much terrestrial ecosystem emissions depend on vegetation cover, as opposed to physical climate (temperature and rainfall).
Parameters for the Pliocene model came from H.J. Dawsett’s Pliocene Research, Interpretation and Synoptic Mapping initiative (PRISM3) data set. These parameters included the lush vegetation cover and topography discussed above, as well as sea surface temperature and sea ice. The chemical composition of the atmosphere was modeled as a function of possible mid-Pliocene methane concentrations since the exact concentration of methane was not known. Meanwhile, the atmospheric boundary conditions for the preindustrial model were based on those of the 1750s, a period before significant human interference had taken place. Sea temperature and ice data for this model reflected data from the years 1876-85, and the vegetation cover was approximated by the standard atlas-based distribution of natural vegetation in the 1750s. Lacking much of the verdancy of the warmer, wetter Pliocene Epoch, the preindustrial Earth had more deserts and semiarid regions throughout Africa, Central Australia, and the Arabian Peninsula. The fire-prone grasslands and savannas that had proliferated on Pliocene Earth had shrunk by the time of the preindustrial period.
Unger and Yue found that the difference in vegetation was key for explaining differences in atmospheric chemistry between the mid-Pliocene and preindustrial periods. The greater abundance of tropical savanna and deciduous biomes in the mid-Pliocene led to more natural emissions from plant life and wildfires. This in turn caused tropospheric ozone and aerosol precursors to persist at levels between 50% and 100% higher than they would in the preindustrial period. According to Unger’s paper, this difference in chemistry-climate was hugely important, accounting for “a net global warming that is +30–250% of the carbon dioxide effect and a net aerosol global cooling that masks 15–100% of the carbon dioxide effect.”
Words of Warning
Pioneering an understanding in the science world of how vegetation creates non-carbon dioxide forcings on the climate is not enough for Unger, who is equally compelled to bring the issue to the public sphere. Her work is especially relevant now as international climate summits, like the one held by the United Nations in New York this fall, explore the possibility of using reforestation to combat climate change. “Trees are magnificent, important things,” Unger told me, but not necessarily the answer to our climate-related problems. Expensive reforestation programs might actually increase the global temperature, both by absorbing hot sunlight with their dark green leaves and by releasing aerosols and precursors into the atmosphere. Additionally, as Unger explained, “climate science doesn’t yet [fully] understand the net effects of tropical, temperate and boreal forests on global climate.”
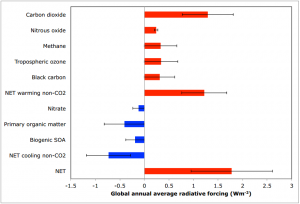
Unger’s greatest certainty lies in the fact that “the U.N. process needs to have more of a focus on fossil fuel CO2 emissions.” As she put it, we do understand “that reducing [these] emissions has a direct and unambiguous impact on the global average temperature.”
Sponsored by wealthy mid-Latitude countries that would rather plant trees than risk industry, reforestation efforts at this point may be mere token gestures. Only cutting back greenhouse gas emissions, costly as that might be, is certain to change the climate for the better. Unger knows it, and she wants us to know it, too: when it comes to fighting climate change, token plantings are not enough.
Recommended Readings:
- Arneth, Almut. “Clean the Air, Heat the Planet?” Science 326, no. 5953 (2009): 672-73.
- Beerling, David. The Emerald Planet: How Plants Changed Earth’s History. Oxford: Oxford University Press, 2007.
- Bowman, David M. J. S. “Fire in the Earth System.” Science 324, no. 5926 (2009): 481-84.
- Fedorov, A. V. “Patterns and Mechanisms of Early Pliocene Warmth.” Nature 496 (2013): 43-49.
- Unger, Nadine. “Global Climate Forcing by Criteria Air Pollutants.” Annual Review of Environment and Resources 37 (2012). annualreviews.org.
About the Author: Hayley Kolding is a sophomore English major in Saybrook College. She has also written for The New Journal and the Yale Journal of Public Health.
Acknowledgements: The author would like to thank Dr. Nadine Unger for graciously sitting for an interview, and for fact-checking this article.