Yale Professor of Electrical Engineering Roman Kuc has answered an important question regarding the ability of a bat to detect motionless prey. For more than 25 years, Kuc has been exploring the world of sonar at the Intelligent Sensors Laboratory, pioneering exploration even as many other researchers, disgruntled with early failures of sonar technology for robot motion, have turned to focus on camera vision. He has taken a keen interest in investigating bats, visiting several of the hundred or so bat laboratories around the world. During the 2000s, he oversaw progress of the EU-sanctioned project ChiRoPing (so named for the bat order Chiropetra, the interest in application to robots, and the audible “ping” of sonar scans), which analyzed bat motion and perception with the goal of creating more effective perception systems. Inspired by an observation from this project, he published a paper co-authored with his son Victor that shed light on the pivotal question of bat perception this September.

In bat echolocation, a bat emits either a continuous sonar signal of a constant frequency, a swept frequency that falls from a higher to lower frequency or a mixture of the two. This signal departs from the area of the bat’s mouth, bounces off the surroundings, and then returns to the often-graded pinna of the bat’s ears to be instantaneously processed. Since the mid-twentieth century, bats have been understood to make use of Doppler shifting to identify prey. For moving objects, the Doppler shift of the returning signal can be used to identify the direction in which the body is moving. A lower frequency than emitted signifies a fleeing body, and a higher frequency signifies motion towards the bat. Prey that is motionless, however, would not Doppler shift sonar echoes, and if silent, could not be separately heard via chirps or squeaks. Such prey would then be lost in the clutter of background.. In observing a video released by the ChiRoPing project, however, Kuc speculated that bats do in fact identify such uncooperative prey by sonar signals through induced movement.
In a slow-motion video released from ChiRoPing, a bat approaches a silent and motionless dragonfly, hovers above it to cause the dragonfly’s wings to flutter, and then grabs and eats its prey. Kuc and Victor suspected that air from the bat’s movements induced the dragonfly’s wing motion that subsequently alerted the bat to the presence of food. They built a biomimetic model to test whether enough information was provided from the bat’s hovering to reasonably identify the dragonfly’s presence. Their experimental model used a modified sonar sensor that could transmit and receive sonar, a sample dragonfly from the Peabody Museum placed upon a plastic leaf, and a second leaf surface positioned slightly closer to the sonar source as a potential deterrent. An automated air brush simulated air pressure from the bat’s moving wings while hovering above the insect. Kuc found that from a significant distance, it was possible to identify the general location of something unusual such as a protrusion on an otherwise flat surface via echo intensity and return time. Moreover, when the air pressure was calibrated to correspond with the bat’s extended hovering, the induced dragonfly wing motion resulted in an increasing echo return time and diminished intensity from the earlier data reading. Processing these observations would hence alert the nocturnal animal of unique and potentially tasty winged prey hiding in the clutter.
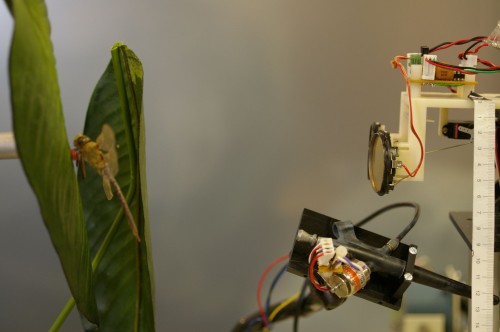
Current studies are investigating if experiments with wingless dragonflies will have similar results and whether moveable structures are necessary to identify silent and soundless objects otherwise hidden in the background. These studies have important implications for sonar technology. Heightened sonar clarity would be very important for underwater mine hunting in the ocean, which the army has been attempting to do with dolphins. It would also prove useful for automated navigation of the ocean, deep ravines, and other unlit areas of the earth.
At the same time, there is also something to be said for simply understanding the bounty of riches provided through the senses. As Kuc exclaims, “All our senses are amazing!” In studying acoustics, he says we have “just another view of the world that might be useful to sonar and might also be helpful in understanding perception in general.”
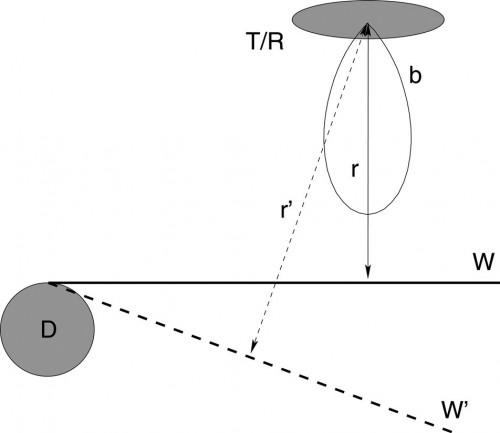