All life exists on a knife’s edge, a delicate balance of the many thousands of biochemical reactions that sustain it. At the cellular level, life is nothing but the proper collection of such chemical processes, all playing out in carefully choreographed sequence, like a well-rehearsed ballet.
But every organism has one big problem: left to their own devices, the chemical reactions necessary for life behave less like practiced dancers and more like an unruly mob. Some reactions proceed with reckless abandon, others unfold slowly, and still many more would never occur at all in the span of human lifetime.
This is where enzymes step in. Enzymes are a class of proteins — large, folded-up molecules — that work to accelerate specific chemical interactions. Within the body, it is up to enzymes to make the reactions run on time. In one extreme example, researchers have determined that a kind of reaction involving molecules called phosphate monoesters would take more than a trillion years to occur on its own. But thanks to an enzyme, it happens in our cells almost 100 times every second.
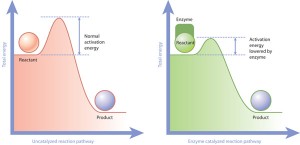
The role of enzymes in reconciling disparate time scales in living organisms makes them an important subject of scientific study. Among those interested in the study of enzymes is Yale University’s Corey Wilson, Assistant Professor of Chemical and Environmental Engineering. Wilson’s research group has focused on exploring the connections between how enzymes work and the temperatures at which they function.
”Enzymes are very sensitive,” Wilson said. They are highly specific in shape; enzymes match the molecules that they act upon just as a glove fits a hand. However, they are not static. The microscopic workings of enzymes involve minute changes of shape, called conformational changes, and an enzyme’s ability to make these changes quickly is essential to its ability to speed up a reaction. Enzymes must find the proper balance between keeping their shape and being able to make conformational changes. Temperature control is the key. Keep an enzyme too cold, and it will be stiff and unable to traverse its proper range of motions. “Too hot,” Wilson explained, “and they will deform.”

Wilson and his team have worked to understand what determines this “Goldilocks” window for each enzyme. So far, their research has allowed them to successfully create designer proteins that function best at a specific, pre-determined temperature. Their work, published this year in the journal Structure, introduces a new range of possibilities for understanding and manipulating the so-called “thermostability” of enzymes.
The team began by modeling protein structures using pre-existing techniques, which do not take temperature into account. They then compared the results of these computer models with experimental data. The gap between model and reality allowed them to elucidate the role played by temperature. Using this new information about enzyme function at different temperatures, Wilson and his team could “rationally design” enzymes that work optimally at temperatures of their choosing.
To do so, they manipulated these protein structures to find more or less stable configurations. “We ask, ‘What is our substrate?’ and then we find another scaffold that will support the same activity,” explained Wilson. The next step was to take these modeled structures and express them as actual proteins in E. coli bacteria. To demonstrate their control of thermostability, they designed and tested 100 different variants of an enzyme called Bacillus subtilis adenylate kinase (bsADK). Normally, bsADK has a very specific thermal niche and “is enzymatically dead within a few degrees outside this temperature,” according to Wilson’s paper. Their new enzymes, however, “occup[ied] a broad distribution of thermostabilities.”
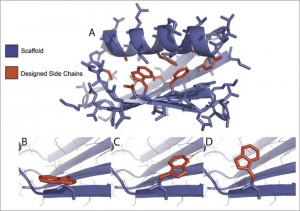
Wilson’s experiments offer scientists both the ability to design enzymes optimized for a particular temperature and a better understanding of enzyme function. The capacity to manufacture custom-made enzymes for use at a specific range of temperatures is exciting because enzymes have numerous applications in both industry and medicine. Wilson sees the possibility of tailoring enzymes to these specific uses. “One exciting example,” he said, “is in biofuel production, where thermostable enzymes are needed to convert cellulose into glucose.”
Wilson also hopes his work will lead to a new emphasis on the importance of environmental conditions — factors like pH and, of course, temperature — for enzyme function. Taking these factors into account will lead to a clearer picture of the highly complex relationship between the structure and function of enzymes. “After all,” said Wilson, “the ultimate test of understanding a system is being able to design it.”