While the term “personalized medicine” may evoke amusing images of a boutique-style doctor’s office, this particular approach to medicine could make an enormous impact on the lives of millions. Currently, most medical treatments are designed to work for the broad majority of the patient population. In contrast, personalized medicine is tailored to particular groups of patients, based on certain genetic characteristics that predict an individual’s response to treatment. Personalized medicine may be the only solution for diseases that are notoriously difficult to treat, such as chronic autoimmune disorders and cancer.
Yale researchers recently discovered a key component involved in the molecular machinery that goes awry multiple diseases. Led by Yale professor of medicine Richard Bucala, the team found a key transcription factor, known as ICBP90, which regulates the activity of a gene involved in inflammation. Although all genes are regulated by many transcription factors, the Yale discovery was notable because this factor regulates a specific region of the gene that is known to be associated with disease. In fact, ICBP90 has already proven to be a promising target for new drugs
The connection between the MIF gene and disease
The gene regulated by this transcription factor codes for a cell-signaling protein in the immune system known as macrophage migration inhibitory factor (MIF). As its namesake suggests, this molecule restricts macrophages—a type of white blood cell— from leaving tissue in the body that is damaged or infected by a pathogen. This protein is highly expressed in the body during inflammation, infection, and injury. As such, the over expression of MIF is frequently implicated in inflammatory diseases such as rheumatoid arthritis, as well as infectious diseases and cancer. In the case of cancer, MIF-related inflammation promotes cell damage, cell proliferation, and tumor growth. Additionally, MIF itself counteracts the expression of a key tumor suppression gene, known as p53.
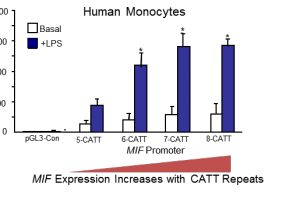
The gene encoding MIF has different genetic variants, or polymorphisms, in the human population. This variation comprises a repeated sequence of four of the building blocks of DNA, or nucleotides: CATT. Individuals can have anywhere from five to eight of these repeats in the MIF gene. Years of genetic and epidemiological studies have established that MIF is minimally expressed when there are five repeats and highly expressed when there are additional repeats. Individuals with this latter polymorphism have significantly worse outcomes when they are also diagnosed with an inflammatory disease. Before scientists discovered MIF, this specific relationship between a polymorphic repeat, increased gene expression, and disease had never been observed.
Until recently, scientists only had one piece of the puzzle, as the regulatory machinery governing MIF was unknown. Using biochemical and proteomic methods to characterize DNA binding proteins by properties such as size or charge, the Yale team explored the CATT locus in more detail. The team succeeded in isolating ICBP90, the transcription factor that binds to this promoter region of the MIF gene. Further experiments revealed that it up-regulates MIF expression, increasing how often the gene is transcribed into RNA. With more CATT repeats, ICBP90 binds more strongly and efficiently to MIF, which in turn elevates expression levels of this gene.
On the road to developing drugs directed at MIF
With these pieces of information, Bucala’s team is now armed with all the necessary components for developing a personalized drug that modulates the expression of MIF. They know that high MIF expression contributes to and accelerates disease. They have a target for their drug—ICBP90—that is known to regulate MIF. Finally, there is a known population of patients with high expression levels of MIF—a high risk phenotype—who could benefit from a drug.
“The idea is you could identify small molecules that interfere with the action of ICBP, [that is] interfere with its ability to bind CATT, “Bucala said. “You could really identify a very specific drug that would allow you to selectively treat diseases of high MIF expression or treat people with a high MIF expression allele.”
Bucala’s lab is currently developing a functional test, or screen, to identify small molecules, such as a short pieces of regulatory RNA called small hairpin RNA (shRNA), that inhibit the function of ICBP90. Bucala hopes that one of these molecules eventually will be transformed into a life-saving drug.
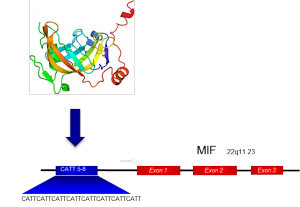
This work dovetails with the lab’s existing efforts to develop anti-MIF drugs. Prior to the discovery of ICBP90, the researchers had developed an anti-MIF antibody that is now in Phase II clinical trials in ovarian and colorectal cancer patients. These antibodies bind to the MIF protein itself and render it nonfunctional. An ICBP90-directed drug molecule, in contrast, would down-regulate the disease-susceptibility gene before the MIF protein is produced, because it targets one of the gene’s transcription factors. Bucala said that such a drug may also have a lower cost and potentially a smaller oral dose. Nonetheless, work on the anti-MIF treatment has made much progress: Bucala founded a Yale start-up, known as MIFCOR, to develop additional clinical applications for MIF.
Moreover, development of the anti-MIF antibody has provided the team with insights for approaching anti-ICBP90 drug development. According to Bucala, one the most challenging facets of drug development is toxicity; most drugs fail the first phase of clinical testing because they do more harm than good for a patient. Data from such Phase 1 trials of the anti-MIF antibody last year demonstrated that there was no maximally tolerated dose for the drug in human subjects. This is a promising sign for both anti-MIF and anti-ICBP90 drug development because it suggests that the team’s intended goal—a significant reduction in MIF protein— will not result in directly damaging secondary effects in patients.
With these encouraging signs, the first approved anti-MIF antibody drugs could reach patients in the next few years and pave the way for the development of more specific ICBP90 drugs. The range of diseases that are impacted by the discovery of ICBP90 may expand, as well, given additional studies. Lin Leng Ph. D., a senior research scientist in medicine on the Yale team, noted that gene regulation is a two-way street: severe under expression of MIF can be just as harmful as over expression. Studies have suggested that the MIF protein has beneficial functions that could be coupled to treatment for a variety of conditions, including one form of heart injury. As such, molecules promoting ICBP90’s activity may also prove to be useful drugs. Combined, studies about the functions of ICBP90 and MIF promise to accelerate the development of personalized medicine for patients.