Membrane fusion, or the process of joining two lipid bilayers into a single lipid bilayer, is an important cellular function carried out everyday by the human body. This biological phenomenon, for example, provides T-cells the ability to engulf bacteria through the process of phagocytosis, an important player in the human immune system response. More generally, membrane fusion tasks include fusion of pathogens with host cells, fusion of eukaryotic cells, and intracellular fusion of organelles. A recent Yale study led by Dr. Frederic Pincet, Associate Research Scientist at Yale School of Medicine, has shed a light on this fascinating process.
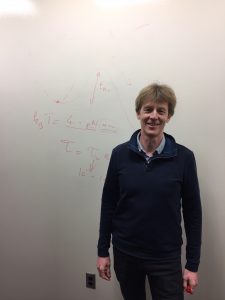
Like most biochemical processes, membrane fusion requires a certain amount of energy, known as the activation energy, in order to run to completion. Pincet notes that early estimates from scientists in the 1990’s put the activation energy for the spontaneous fusion of membranes at over 100 kBT (unit of kBT measures the product of Boltzmann’s constant, k, and temperature).
Since the early estimates, models have predicted a wide range of activation energies for this process, with estimates ranging from 20 kBT to 150 kBT. Dr. Pincet, however, pointed out that the high estimates failed to account for the fact that fusion events are known to occur spontaneously. With respect to the original high estimates, “[Those reaction times] are a lot longer than the age of the universe. Fusion would never happen on its own,” Pincet said. The optimal membrane fusion activation energy prevents spontaneous fusion from occurring too rapidly yet can still be overcome by specific fusion proteins, such as soluble N-ethylmaleimide-sensitive factor attachment protein receptors (SNAREs). Therefore, the Rothman Lab at Yale set out to measure the activation energy of spontaneous membrane fusion.
The experimental procedure, known as FRET (Fluorescence Resonance Energy Transfer), involves fusing a ratio of blank vesicles to fluorescent vesicles and measuring the fluorescence intensity of green and red dyes with respect to time. The fluorescent vesicle contains both a red and green dye, and when the green dye is excited, energy transfer between the two dyes causes the red dye to become excited and emit a red fluorescence. If the two dyes are too far apart, however, the energy transfer is not present, and only the green fluorescence is seen. By measuring fluorescent intensity with respect to time, the reaction rate can be determined.
The researchers found that typical lipid vesicles were found to slowly and spontaneously fully fuse with activation energies of ∼30 kBT. To Dr. Pincet, this value was reasonable. The energy is sufficient to prevent membranes from spontaneous fusion, but if energy is brought in with SNAREs, the membrane will fuse 100% when it is triggered. “If a SNARE brings 5 kBT, the energy needed comes down to around 25 kBT, and since time is exponentially related to energy, this reduces the time of the reaction very quickly. It goes from a timescale of days to the timescale of milliseconds needed for neurotransmission,” Pincet said. For such an important biological process, it is apparent that our body has optimized membrane fusion in order to prevent wasteful spontaneous fusions and to allow proteins to control the rate of reaction by lowering the activation energy.
This finding has tremendous implications in the fields of neuroscience. In neural transmission, where speed of signals is of utmost importance, optimizing the rate of the reaction by lowering the activation energy and raising reaction velocity is a neat feature that the activation energy threshold of 30 kBT provides.
Featured Image: This image shows a SNARE protein assisting in the process of membrane fusion. SNAREs are essential to membrane fusion as they bring the requisite energy needed to lower the activation energy of the reaction and allow the biological event to be carried out in a much smaller timescale. Image courtesy of Dr. Frederic Pincet.