Twitch and swim, slide and glide. Some of the wildest ways of dancing through life are exhibited by the tiniest of organisms — bacteria. Some bacteria are heavily equipped movers, armed with powerful propellers called flagella that rotate rapidly and thrust the organism forward. Others opt for a more delicate route, extending long, spidery legs called pili that reach out and then retract, pulling the organism forward. And still others simply slide themselves through the world, secreting wetting agents that allow them to slip along.
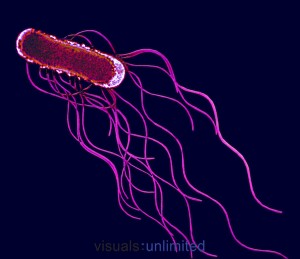
This colorful repertoire of bacterial movements is not simply for show. “Bacteria must find food, and they must escape toxic environments. Being able to move is a huge help,” said Eduardo Groisman, Yale professor of microbial pathogenesis.
In a recent study, Groisman and his team identified a new mode of surface motility in the bacterium Salmonella enterica. Salmonella, notorious for inducing nasty bouts of food poisoning and prompting parental warnings of “do not eat the cookie dough,” usually pushes itself forward with flagella as motors. These powerful propellers cost a lot of energy, and are under tight regulation: When the key nutrient magnesium dips below critical levels in the bacterium’s immediate surrounding environment, a regulatory system kicks in and shuts down the bacterium’s flagella. Scientists previously thought that the bacteria would be unable to move in this low-magnesium condition. But Groisman’s lab recently reported that Salmonella is actually able to move in a manner that does not involve flagella at all. The discovery provides exciting insight into the lifestyle of Salmonella, as well as into the rich diversity of choreography in the bacterial world.
All about magnesium
All cells, from bacteria to those that comprise our own bodies, are crisscrossed with a web of regulatory systems that respond to constantly changing environments. Groisman’s exploration of bacterial motility was borne out of a long-standing interest in the PhoP/PhoQ regulatory system in Salmonella, an intricate regulatory pathway that, among its many roles, helps monitor the motion of flagellar motors.
A major trigger for the PhoP/PhoQ system is the nutrient magnesium. “Magnesium is crucial for cells,” said Sun-Yang Park, postdoctoral fellow and lead author of the study. It is important for maintaining membrane structure, neutralizing the negative charges of the “energy currency” molecule ATP, and stabilizing DNA and RNA. When magnesium levels outside the cell plummet, the change is sensed by the PhoP/PhoQ system, which controls the expression of a large number of genes. For example, the system can stifle flagellar motility genes in the absence of magnesium.
Just as we would not buy a Lamborghini if we are struggling to make ends meet, bacteria forego costly ways of getting around when times are tough. Shutting off energy-expensive flagella when magnesium levels are low allows the bacterium to conserve energy when it is facing a stressed, nutrient-depleted environment. And if the Salmonella is invading a host, loss of flagella is not too problematic, anyway. Motility is inconsequential if the bacterium is tightly trapped within the vacuole of a macrophage, a cell of the immune system. Since flagella are often easily recognized by host immune systems, it may actually be beneficial for the bacterium to deactivate these motors.
Still, though blocking expression of flagella under conditions of low magnesium helps conserve energy, it seems counterintuitive to restrict motion when the bacterium could be moving around in search of nutrients. Could an alternate mode of motility be triggered when magnesium is depleted from a bacterial cell’s surroundings? Perhaps a method of getting around that is not as energetically expensive as are flagella?
A surprise in the lab
One day, as Park was spotting bacteria on an agarose plate with low magnesium, she noticed something unexpected: wild-type Salmonella enterica bacteria she had been growing in an environment with low magnesium seemed, remarkably, to be migrating. This was strange because under conditions of low magnesium, the PhoP/PhoQ system should have shut down the rotation of flagella, the only known mode of Salmonella movement.
Indeed, whatever was inducing this mysterious motility, it certainly was not dependent on flagella. When Park knocked out genes essential for flagellar function, the bacteria continued to migrate unhindered. Similar experiments demonstrated that the motility was not caused by pili, the spindly appendages usually required for twitching motility. These findings were unlike anything the researchers had ever seen.
Park’s experiments further showed that mutants with the PhoP/PhoQ system knocked out are immobile under low magnesium. It seems that the regulatory system, the very pathway that turns off flagella-mediated motility when magnesium is depleted, simultaneously turns on this mysterious alternate mechanism to keep the bacteria moving.
Park went on to identify three PhoP-activated genes that are essential for motility under low magnesium surroundings: mgtA, mgtC, and pagM. She determined that mgtA and mgtC control the expression of the pagM gene, which encodes a small protein of unknown function that seems to mediate motility in some way. Despite this remaining ambiguity, the solution to the unexpected migration was coming into clearer focus.
Moving as a team
So how does the PagM protein promote motility in Salmonella? The details of the mechanism are still fuzzy, but Park said she has some clues. She believes that PagM or another protein could be attached to the outer surface of the bacterial cell membrane to help promote motility through a mechanism that does not require energy — a stark contrast to energy-guzzling flagella. Indeed, treatment of the bacteria with proteases, enzymes that chew up any proteins fastened to the cell’s outer surfaces, successfully abolished motility, suggesting that a surface protein is involved.
Additionally, it seems that the bacteria are not simply moving in a solitary fashion —rather, pagM-mediated migration is a group effort. When immobile pagM mutants were incubated with mobile pagM-expressing bacteria, the mobile bacteria were able to confer motility to the pagM mutants, essentially pulling along their motionless friends like baggage. This group behavior model lends support to the sliding mechanism of motility hypothesis: Surface proteins expressed on one bacterium could enable immobile bacterial neighbors to slip along as well.
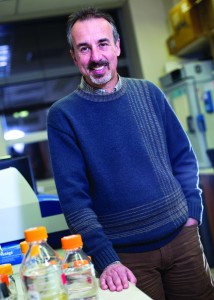
Groisman noted that the evolution of this behavior involves the emergence of “cheaters,” or organisms that take advantage of conditions created by others so that they do not have to generate those conditions themselves. Essentially, immobile cheaters save the energy of producing machinery needed to move, instead simply hopping a ride with their neighbor. In this way, Groisman explained, cheaters benefit. They use less energy to migrate in the same way as their hardworking peers — a less than friendly arrangement.
Getting to know bacteria
Cheaters or not, those that reap the benefits of pagM-mediated motility have a distinct edge. Experiments showed that pagM-expressing strains were able to proliferate much more successfully than pagM mutants lacking the system in low magnesium conditions. “This mode of motility could allow bacteria to seek out environments with more nutrients and escape toxic waste products, offering a growth advantage,” Park said.
Additionally, this form of movement could help Salmonella find its way without relying on a host, such as in soil environments with low levels of magnesium. This glimpse into the life of Salmonella outside our bodies comes as a welcome, important contribution to the field of microbiology. “What’s so remarkable about this finding is that it provides insight into aspects of Salmonella’s lifestyle that we know little about. We know very little, for example, about how Salmonella survives in soil. This discovery provides needed insight into how Salmonella persists in non-host environments,” Groisman said.
Moving forward, Groisman and Park hope to clarify the mechanism of motility by using newly acquired antibodies to localize the PagM protein. They also plan to investigate potential protein partners that may interact with PagM.
Even beyond the world of Salmonella, Groisman emphasized that this discovery reveals a new way in which bacteria move, especially in search of nutrients. Having identified another mechanism of motility, we are one step closer to understanding how bacteria dance. “This may very well be a new way a microorganism can move,” Groisman said. “It is like nothing that has been described before.”
About the Author: Malini Gandhi is a sophomore molecular, cellular, and developmental biology major in Morse College. Starting this summer, she will be working in Dr. Akiko Iwasaki’s lab studying interferon stimulated genes and HIV. She is also passionate about creative writing.
Further Reading:
Adams et al. “Proteomic detection of PhoPQ and acid-mediated repression of Salmonella motility.” Proteomics. 1.4 (2001): 597-607.
Harshey, RM. “Bacterial motility on a surface: many ways to a common goal.” Annual Review of Microbiology 57 (2003): 249- 273.
Groisman, E.A. “The Pleitropic Two-Component Regulatory System PhoP-PhoQ.” Journal of Bacteriology 183.6 (2001): 1835-1842.
Park et al. “Signal-specific temporal response by the Salmonella PhoP/PhoQ regulatory system.” Molecular Microbiology. 91.1 (2014): 135-144.
Cover Image: Art by Lindsey Stavola.
Acknowledgements: The author would like to thank Dr. Groisman and Dr. Park for their time and for enthusiastically discussing their research.