At first glance, nature and technology may seem like opposites. Leaves stand in contrast to circuits, birds to airplanes, and mountains to skyscrapers. But technology has a history of taking cues from nature. Velcro was inspired by burdock burrs, while aircraft were modeled after bird wings. The Shinkansen Bullet Train was constructed with the kingfisher’s beak in mind. A closer lens on nature unlocks tremendous potential for technological innovation, and plant cells are no exception.
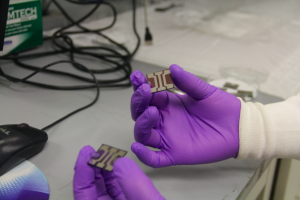
Photo by Genevieve Sertic.
Yale researchers are now looking to plant cells in order to improve the design of solar power, touted as a carbon-free alternative energy source. At the heart of solar power are solar cells, which, like plant cells, aim to absorb sunlight and turn it into a useable form of energy. André Taylor, associate professor of chemical and environmental engineering, and Tenghooi Goh, graduate student in the School of Engineering and Applied Science, worked with their team to develop an organic solar cell that mimics the functions of a plant cell.
Most solar power today relies on silicon solar cells, which do not precisely parallel plant cells. When sunlight hits a silicon solar cell, an electron jumps across the material and moves through a wire to generate electricity. Plant cells instead take the light energy and transfer it to a protein through a chemical process. Organic solar cells are relatively new in the field of solar energy. There are many different types, but generally speaking, organic solar cells are lighter, cost less, and have more environmentally-friendly manufacturing processes than their traditional silicon counterparts.
At this point, the choice of a solar cell probably seems obvious. But organic solar cells come with one major drawback: efficiency. Solar cell efficiency refers to the amount of electricity generated relative to the input of sunlight energy. While silicon cells have achieved efficiencies of more than 20 percent, organic cells are lagging behind.
Taylor and his team sought to increase this efficiency while maintaining the advantages of organic solar cells. They blended together two polymers with complementary properties, aligning them to make them compatible. Together, these polymers can absorb light from much of the visible spectrum, which explains their greater combined efficiency: The Yale researchers managed to increase efficiency of this particular type of solar cell by almost 25 percent.
The key to better solar energy, as it turns out, lies in nature.
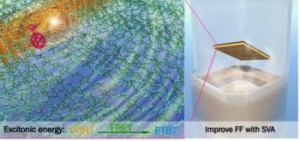
A three-part design
To turn light energy into electrical energy, organic solar cells need a material that gives up an electron — a donor — and a material that takes that electron — an acceptor. However, the donor polymer can only absorb a certain range of light wavelengths. Wavelengths outside of this range are wasted. The recent development from Yale scientists allows an organic solar cell to absorb a wider range: Adding another donor that accepts a different but complementary range of light wavelengths gets at the efficiency problem directly.
These new types of solar cells are called ternary cells, and they have three components: two donors, one acceptor. Unfortunately, more often than not, the two donors conflict with each other and lower the overall efficiency of energy conversion.
Polymers P3HT and PTB7 are two such incompatible donors. They align in completely different directions, with P3HT standing vertically and PTB7 lying horizontally. In poorly-aligned structures, charge recombination occurs, wherein an electron meant to generate electricity is reabsorbed into a hole in the material, or a place where an electron could exist but does not.
But not all hope was lost for P3HT and PTB7. Taylor’s team noticed that the wavelengths of light absorbed by the polymers are in fact complementary — P3HT takes in blue-green light, while PTB7 is best at absorbing light in the yellow-red spectrum. Overcoming their incompatibility would allow for a much more efficient ternary cell, and this is exactly what Taylor’s team set out to do.
Finding agreement in incompatibility
In order to reduce the interference between the two donor polymers, the team focused on a couple methods, including Förster resonance energy transfer (FRET). FRET is a mechanism by which two light-sensitive molecules, or chromophores, transmit energy. This process helps primarily in biological studies to trace proteins as they travel through cells. It is also one of the primary mechanisms in energy conversion within a plant cell, and in fact, is one of the reasons that leaves are so efficient in converting sunlight into chemical energy. FRET is not a topic normally brought up when discussing solar technology, however. “It’s been heavily used in biology, but never in polymer solar cells,” Taylor said.
In this study, the researchers focused on FRET between their two chromophores, polymers P3HT and PTB7. Individually, the efficiency of each polymer is not particularly powerful. However, combining the polymers facilitates FRET and allows them to complement each other, resulting in an efficiency of 8.2 percent — quite high for a ternary organic solar cell.
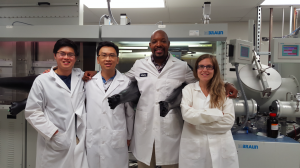
Photo by Genevieve Sertic.
Other research groups have also used various polymers in conjunction, but never in a way that forces the polymers to interact. Taylor’s team combined P3HT and PTB7 and created a collaboration, where one polymer picks up emissions from the other. “We’re the first group to show that you can actually put these components, these multiple donors, together, and have them act synergistically,” Taylor said. The polymers are complementary — one can recover lost energy from the other, and together, they can take in a much wider range of light. This was among the most pivotal findings in the Yale study.
To improve efficiency further, the researchers next focused on adjusting the incompatible alignment of the polymers. Electron flow is impeded between P3HT and PTB7. “If organics align in a conflicting way, they will not allow electrons to flow in a favorable direction,” Goh said. A second method the team used, a technique called solvent vapor annealing, can fix that. The researchers exposed the solar films containing the incompatible polymers to vapor to help the structures relax and smooth out. With this technique on top of the special attention to FRET, the organic solar cells achieved a remarkable efficiency of 8.7 percent.
Strategizing for the future
This research on new organic solar cells is not only significant because of increased efficiency. It also describes an innovative process for overcoming mechanical difficulties within organic solar cell materials. Even after Taylor’s improvements to ternary cells, organic-based solar power is not matching the efficiency level of silicon-based solar power. But using their methods as a launching pad, there is great potential to increase efficiency of organic solar cells even further in the future.
“As people are developing newer polymers, they can use this study as a road map to create higher-efficiency devices,” Taylor said.
This study shows that polymers labeled as incompatible can be re-engineered to complement each other and to increase solar cell efficiency. It also illustrates that nature’s answers to technological challenges are as relevant as ever.
Beyond their basic function of turning sunlight into useable energy, plant cells and solar cells might not seem related at first. Plant cells convert sunlight into chemical energy, while solar cells convert sunlight into electricity. But the mechanisms by which plant cells absorb a wide range of solar radiation are, as it turns out, readily applicable to the choice of polymers in organic solar cells. In fact, plant cells provide a model that the Yale group found to be incredibly helpful. The story of solar cells inspired by plant cells introduces not only new technology, but a new way of thinking about solar cell efficiency that reflects our natural world.
About the Author: Genevieve Sertic is a sophomore prospective Electrical Engineering major and Energy Studies Undergraduate Scholar in Pierson College. She is a Copy Editor for the Yale Scientific Magazine and works through Project Bright to promote solar power on Yale’s campus.
Acknowledgements: The author would like to thank Dr. André Taylor and Tenghooi Goh for their time and enthusiasm about their research.
Further Reading: Huang, Jing-Shun et al. 2013. “Polymer bulk heterojunction solar cells employing Förster resonance energy transfer.” Nature Photonics 7: 479-485. doi: 10.1038/nphoton.2013.82
Cover Image: Art by Chanthia Ma.