“We talk about a pre-antibiotic era and an antibiotic era. If we’re not careful, we will soon be in a post-antibiotic era. And, in fact, for some patients and some microbes, we are already there.”
–Tom Frieden, CDC Director
Scientists predict that without a major pharmacological breakthrough, deaths from antibiotic resistant bacteria will surpass cancer deaths by the year 2050. However, thanks to a team from the Melbourne School of Engineering, that breakthrough may be a bit closer. The team designed tiny, star-shaped polymers that were highly effective against multiple types of bacteria without allowing resistance development. Though more testing and development is required, scientists are hopeful that these polymers could avert a deadly global health crisis.
Just as humans can build up tolerance to poisons by exposing themselves to incremental, sub-lethal doses over time, bacterial colonies can develop resistance to antibiotic drugs when exposed to non-lethal quantities. Although the biological processes are different, the result is the same: substances that would normally prove lethal suddenly fail to have an effect. In the case of antibiotic-resistant bacteria, this can mean infections and diseases that were once easily treated suddenly become deadly. Without antibiotics, even routine procedures like kidney dialysis, appendectomies, hip replacements, and biopsies would suddenly carry extremely high risk.
Manisha Juthani-Mehta, an infectious diseases physician at the Yale School of Medicine, notes that drug-resistant bacteria have been around for decades, but modern medicine is exacerbating their proliferation. “We have far more [drug-resistant bacteria] now with prevalent overuse of antibiotics,” she said. The agricultural industry is by far the largest culprit of overuse, purchasing 80% of all antibiotics sold in the United States every year. The drugs help promote plant and livestock growth and protect crops and animals from diseases. While these uses are important, they are not so crucial that cutting back would be impossible. Overuse drastically increases the rate at which drug-resistant bacteria develop and spread in the environment and in the food supply. Nursing homes and hospitals are other major breeding grounds for antibiotic resistant bacteria. “In nursing homes, infections are commonly suspected and antibiotics are frequently prescribed. Older nursing home residents have multiple medical problems and are often exposed to multiple rounds of antibiotics” said Juthani, whose expertise involves infections in older adults. Although scientists and government agencies have encouraged farmers and medical professionals to limit antibiotic use, no strict regulations have been passed.
For some infections, we are running out of treatment options. “We are more often stuck using very toxic, old antibiotics because we have no choice,” said Juthani. In some cases, even these last resorts are failing. Each time a bacterial infection becomes resistant to a particular drug, physicians can only hope that a new, more effective drug will be developed. Unfortunately, because bacteria generally develop resistance to a drug very quickly and thereby render it obsolete, antibiotic development is not profitable for pharmaceutical companies. “There have only been one or two new antibiotics developed in the last 30 years,” said Greg Qiao from the University of Melbourne in a Science Daily article.
That’s where the ‘stars’ come in. A team of Australian scientists—including Qiao, Eric Reynolds, and PhD candidate Shu Lam—recently published a paper in Nature Microbiology describing a promising alternative technology to combat multidrug-resistant bacteria. Instead of designing a traditional chemical drug treatment, the team developed what they call “structurally nanoengineered antimicrobial peptide polymers,” or SNAPPs for short. The researchers were inspired by natural antimicrobial peptides, which are small proteins that play important roles in the immune systems of many organisms. Naturally occurring antimicrobial peptides cannot be used in clinical settings because they are often toxic to mammalian cells, but Lam and her team wanted to use them as a model for designing a powerful and safe antibiotic agent.
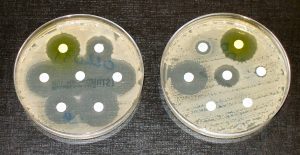
The scientists meticulously designed the polymers, down to the level of the individual building blocks—amino acids—that would make up the peptides. Out of the many amino acids available to them, the scientists chose lysine and valine. Lysine is a positively charged cation, and was selected because cationic peptides were already known to exhibit antimicrobial activity. Valine, on the other hand, is uncharged and therefore hydrophobic, meaning it does not interact favorably with water or other polar molecules. Since hydrophobic materials interact favorably with other hydrophobic materials, valine’s hydrophobicity enables the SNAPPs to infiltrate the cell membrane, which is also mostly hydrophobic. Instead of just creating long chains of amino acids or allowing the polymers to self-assemble, the researchers attached groups of 16 or 32 chains to a multifunctional core, which served to promote water solubility and create the characteristic star shape. They hypothesize that the star shape optimizes functionality because it promotes peptide aggregation and localized charge concentration, which leads to more effective ionic interactions with bacterial membranes.
After designing and successfully producing the polymers, the researchers assessed the activity of the SNAPPs against different species of bacteria. The SNAPPs were active against all bacterial species but were especially effective against Gram-negative bacteria like E. coli. Gram-negative bacteria are characterized by an outer membrane that normally acts as a highly impermeable barrier, but the researchers discovered that the SNAPPs could penetrate this membrane since they have a high affinity for specific molecules found on it. The treatment was equally effective against antibiotic-resistant and susceptible strains of bacteria. The effectiveness of SNAPPs against Gram-negative bacteria is especially important because no antibiotic drugs currently under development are effective against Gram-negative infections.
Before testing SNAPPs in living organisms, the researchers first performed a biocompatibility assay to ensure that the polymers would not attack mammalian cells. By incubating the polymers with sheep’s blood and measuring death rates of blood cells, the scientists determined that SNAPPs exhibit very low toxicity, even at concentrations 100 times higher than what is required to kill bacteria. After confirming biocompatibility, they tested the effectiveness of SNAPPs by treating mice with rampant bacterial infections. The results were very promising—all mice treated with SNAPPs lived, compared to only twenty percent of the untreated mice. In addition, SNAPP treatment enhanced the ability of white blood cells to infiltrate infected tissues, a benefit not displayed by mice treated with traditional antibiotics.
The SNAPPs have multiple mechanisms of killing cells, making it more difficult for bacteria to develop resistance against them. The polymers’ partially hydrophobic composition allows them to infiltrate the membrane, but once they have done so, the positively charged amino acids disrupt membrane integrity and prevent regulation of ion flow. The star-shaped polymers can even aggregate and rip apart the membrane. The SNAPPs may also trigger the cellular processes that induce apoptosis, or cell suicide. All these mechanisms of antibiotic action are impressive individually, but when combined in a single molecule they are incredibly powerful and difficult for bacteria to fight. Even after exposing 600 generations of bacteria to low concentrations of SNAPPs, the researchers could not detect bacterial resistance to the treatment. These results show great promise for SNAPPs as a long-term solution to the rise of superbugs.
To bring treatments like SNAPPs into regular use, more research, development, and eventually clinical trials are needed. Although many industries and the public still fail to heed scientists’ warnings about antimicrobial resistance, governments and research institutions are starting to focus on the war against drug-resistant bacteria. On September 21st, the United Nations held a summit on antimicrobial resistance and concluded that all countries must formulate a plan to combat it. At the beginning of October, the CDC announced that a research team at the Yale School of Public Health—along with 33 other teams—will receive funding as part of a $14 million dollar effort to research antibiotic resistance. Hopefully, this collaboration between scientists and governments will allow SNAPPs—and perhaps other new technologies—to better aid in humanity’s battle against antibiotic resistant bacteria.