Photography by Michelle So
From ocean waters to soil, and from hot springs to human microbiomes, tiny battles between bacteria and viruses are constantly unfolding. These battles start when bacteriophages, the viruses that infect bacteria, bind to receptors on a bacterial host cell. In a process known as lytic replication, the phage injects its genome into the bacterium, using the host’s resources to create proteins that are assembled into more phages. This eventually causes the cell to rupture and die, releasing the newly created phages into the world.
In their simplest form, phages consist of a viral genome encased in a protein shell. Some types of phages have spider-like “legs” extending from their main structure, giving them an otherworldly appearance reminiscent of a robot or spaceship. These microscopic oddities appear in nearly every environment on Earth.
But phages are more than just a novelty. Since they have spent billions of years evolving to destroy bacteria, they could be a potent weapon in the fight against antibiotic-resistant germs. Scientists have been studying phages for more than a century, seeking to understand their attack strategies. However, researchers have long been limited by phages’ tiny size, which are often orders of magnitude smaller than the bacteria they target. In order to understand phages, scientists have to see them first.
In a recent study published in Proceedings of the National Academy of Sciences, researchers from the Yale Quantitative Biology Institute—an inter-departmental collaboration between biological and physical scientists—described a new assay method for dynamically visualizing these microscopic interactions with improved resolution.
Seeing is Believing
The traditional method of visualizing bacteria-phage interactions requires mixing bacteria and phages together in a flask, periodically taking small samples of the mixture, and measuring how many phages have not yet attached to bacteria at each point in time. This process, known as a classical adsorption assay, allows researchers to calculate a phage adsorption rate—how quickly phages attach to bacterial cells over time. The downsides to this classic assay, however, are that it is time-consuming, labor-intensive, and only provides estimates of the average adsorption rates across entire populations of bacteria.
The team of Yale researchers developed a Microscopic Phage Adsorption (MPA) assay to quantify bacteria-phage interactions at the level of individual viruses. The first step in the MPA assay is to label phages using a fluorescent dye so that they glow brightly under the microscope. A carpet of non-moving bacterial cells is prepared on a glass slide, providing a stable surface for phages to attach to. Then, the labeled phages are introduced to the bacteria, and researchers can visualize the sample using microscopes that reveal the fluorescent labels. The phages appear as bright spots against the background of bacteria. Using this technique, researchers can record videos of the phages and bacteria in real time, documenting the dynamic movements and individual attachments of phages to bacteria.
“In the traditional approach, where you mix viruses and bacteria together in a flask, you are getting average readouts from millions or billions of viruses and bacteria,” said Jyot Antani, a postdoctoral researcher and first author of this study. “But here, you can actually see what’s going on at a single virus level.” This level of detail is particularly important because previous methods of microscopy could not account for possible variation in dwell time—the length of time a phage spends latched to a singular bacterium—between phages. Each type of phage can only attack one specific bacterial species or strain, and their attack strategies differ. Knowing the differences in dwell time between different phage types may help researchers better understand the strategies that exist in phage ecology and how these strategies influence phage population dynamics.
A New Paradigm
Using mathematical software and the time-lapse videos taken of the phages, Antani and the research team were able to chart the trajectories of individual phages over time. Some phages moved freely, appearing in the microscope’s view for only a short time before drifting away, while others remained relatively fixed in place, staying attached to the same bacteria for the duration of the observation period. By analyzing these movement patterns, the researchers could distinguish between transient and stable phage-bacteria interactions.
“In a biological population, there is always heterogeneity, and this is a way to characterize the heterogeneity in virus attachment to bacteria,” Antani said. Observing the unique behaviors of single viruses reveals variations that ensemble-averaged measurements might obscure, providing a more precise and detailed understanding of virus-bacteria interactions—much like how measuring an individual person’s vital signs offers better health insights than relying on population averages. Antani explained that he performed the phage trajectory analysis with seven different strains of bacteria, whose phages each have different dynamics of interaction. The results of the MPA assay correlate with the traditionally measured adsorption rate. This shows that the MPA assay method is an efficient, accurate alternative to classical methods of phage visualization.
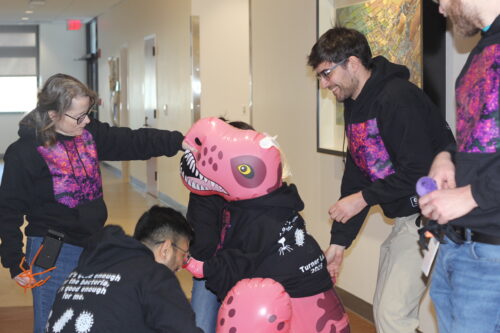
Future Phage Technologies
The targeted use of phages in future technologies has many important biological implications. One of their most emergent uses is in phage therapy, a branch of personalized medicine that uses phages as drug candidates to treat bacterial infections. For instance, through phage therapy, scientists could quickly sample a harmful strain of bacteria in an infected patient and determine the type of phage that would be effective in treating their condition. This type of phage treatment is currently undergoing rigorous testing and clinical trials before it can be used in humans on a wider scale. In the future, as antibiotic-resistant strains of bacteria become more prominent, phage therapy might emerge as a potent alternative.
Another use of phages may be in environmental engineering. Bacteria are found in many natural ecosystems, but they are especially prominent in water systems such as oceans, lakes, and ponds. When bacteria that are harmful to human health end up in reservoirs, they can cause widespread harm. Paul Turner, a professor of ecology and evolutionary biology at Yale who is an author on this study, explained that MPA assays could be used to quickly detect phages in water samples. These phages, in turn, indicate the presence of their bacterial hosts. “If you can imagine this technology being portable, you could go to a lake or reservoir and use it to get a real-time readout of phages and bacteria of interest that might be pathogens in some system,” Turner said. Then, you could test for phages that may destroy those particular pathogens and apply them to the body of water in order to remove harmful bacteria.
The diverse potential uses of phages make them one of the most exciting new areas to explore in biology. Thanks to the MPA assay and its new method of visualizing the microscopic behaviors of phages, researchers can develop a better understanding of the microbial interactions that shape so much of our world. As scientists continue to refine this technology, the insights gained could lead to both medical and environmental applications, harnessing the power of phages to combat bacterial infections and monitor ecosystems. With each advancement, we move closer to unleashing the full potential of these ancient microbial warriors.