Art by Melody Jiang
The universe was born in a fiery explosion, expanding outwards in all directions. For billions of years following the Big Bang, the tug of gravity worked to slow its growth. But then, the unexplainable happened—the universe began to expand at an accelerating rate, driven by an invisible force scientists have termed “dark energy.” Determined to explain this cosmic mystery, over nine hundred researchers from around the world joined forces to create the Dark Energy Spectroscopic Instrument (DESI), the world’s most powerful multi-object spectrograph.
Perched high in the mountains at Kitt Peak National Observatory in Arizona, DESI is now concluding a five-year survey with the ambitious goal of mapping a third of the sky and capturing data about the light spectra emitted from thirty-five million galaxies. Its unprecedented 3D cosmological map will allow scientists to measure the influence of dark energy on the universe’s expansion. Two Yale researchers—Charles Baltay and David Rabinowitz—have played a crucial role in developing DESI’s key technologies, from fiber-optic imaging to advanced data analysis tools.
Baltay, Yale’s Eugene Higgins Professor Emeritus of Physics, describes himself as an experimentalist studying fundamental issues. He began his career in the 1960s, studying strongly decaying particle states, but has more recently shifted towards astrophysics and cosmology, researching dark energy via the study of distant supernova explosion events. “Just about one hundred years ago, we thought we understood classical physics. […] Then along came atomic physics, and atoms did not behave the way we predicted […], so we had to invent a whole new physics called quantum mechanics and relativity,” Baltay said. He suggests that we are at a similar point now as the universe is not doing what we predict it to do. “We have to invent a whole new physics, whether that’s dark energy or a change in general relativity, but it’s a fundamental, basic change in what we understand.” As DESI rapidly collects data, scientists eagerly await insights into the creation and eventual destiny of our universe. Will the universe continue expanding? Or is a more dramatic fate awaiting us—a collapse as violent as the Big Bang?
Blasts from the Past
The fundamental issue of dark energy lies in its intangibility. Unlike ordinary matter and energy, dark energy neither emits nor interacts with light, making its direct observation impossible with current telescopes and instruments. Dark energy should not be confused with dark matter; while both are cosmic and invisible, dark matter pulls matter together, while dark energy pushes it apart.
If dark energy cannot be touched or seen, how do we know it exists? Well, dark energy is our current term, coined by University of Chicago astrophysicist Michael Turner in 1998, for some force pushing the universe to expand faster than it should. Based on our understanding of cosmology at the time, scientists in the nineties expected the expansion of the universe to be slowing down over time as gravity pulled galaxies together. However, this is not currently the case. In fact, by observing supernovae that exploded billions of years ago and calculating the rate at which the galaxies they are in are moving away, scientists have been able to conclude the rate of expansion of the universe is currently increasing. This rate of expansion is now termed the “Hubble constant,” after American astronomer Edwin Hubble, who first discovered that the universe was expanding decades prior.
These calculations have led to the development of a mathematical model for the Big Bang known as the Lambda-CDM model. According to this model, the universe’s expansion is shaped by two forces: cold dark matter (CDM), which interacts weakly with ordinary matter and slows expansion through gravity, and dark energy (Lambda), which drives the universe to expand at an accelerating rate. The expansion of the universe must have been slowing for a time, as galaxies would not have been able to clump together otherwise, but around five billion years ago, dark energy’s repulsive force must have overwhelmed the attractive gravitational interactions between matter.
Using observations from supernovae and light left over from the early years of the universe after the Big Bang, scientists have also been able to accurately estimate the current quantity of dark energy, estimating that it composes up to seventy percent of the energy in the universe. Attempting to reconcile this illusive energy, an enormous part of our universe, with our current cosmological understanding, astrophysicists have developed three primary theories of dark energy’s interaction with classical physics.
The “cosmological constant” or “vacuum energy” theory is the most widely accepted of these conjectures. It argues that dark energy is created by a vacuum of space, in turn generating cosmic acceleration. In contrast, the “evolving dark energy” theory proposes the existence of an unknown universal field that opposes the normal effects of matter and energy, leading to varying quantities of dark energy over eons. The final, aptly titled “alternative gravity” theory suggests that, perhaps, dark energy does not actually exist—rather, Albert Einstein’s theory of general relativity may be missing a piece, causing miscalculations that incorrectly imply the presence of dark energy.
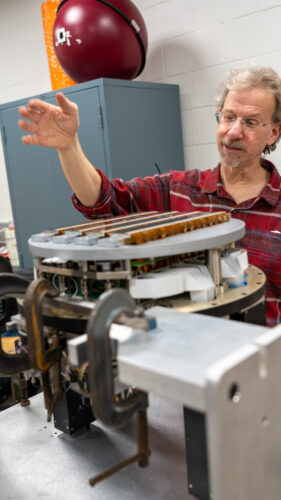
DESI’s Galactic Census
All these differing theories on the nature of dark energy raise the question—how do we measure the invisible? Before DESI can track cosmic expansion—which it accomplishes by following galaxy movement—scientists must first know the 3D distribution of galaxies in space. To accomplish this, DESI measures the influence of dark energy through baryon acoustic oscillations (BAOs) and galaxy redshift-space distortions. BAOs arise out of the composition of the primordial universe: tiny fluctuations in ionized plasma sent pressure waves rippling through a soup of hydrogen and helium nuclei, regular matter. As the universe expanded and cooled, the waves froze in place, creating a characteristic separation of galaxies that act as DESI’s cosmic rulers.
In addition to BAOs, DESI tracks galaxy position by measuring redshift. As light travels from across the expanding universe, its wavelength elongates, shifting towards a lower energy form. For visible light, this process transforms blue light into red. The farther away a galaxy is, the more its light is redshifted, providing a direct link between distance and motion. While the light of typical galaxies may eventually become too faint to detect at extreme distances, DESI looks at quasars, which are very bright galactic cores with black holes at their centers. As their light traverses intergalactic gas clouds, the light spectra become patterned with dark lines indicating various redshifts depending on the clouds’ distances from the quasar. This pattern of absorption lines is known as the Lyman-alpha forest, named such because it originates from the Lyman-alpha transition that electrons can make in hydrogen atoms. Using the distance information encoded in the Lyman-alpha forest, scientists can track BAO and redshift measurements back eleven billion years. To ensure the accuracy of these findings, DESI is the first spectroscopic experiment to perform fully “blinded analysis,” in which the data is concealed from the researchers until the analysis is performed to limit subconscious confirmation bias.
Collecting the precise galaxy positions used in these measurements was made possible by an extensive imaging survey conducted before DESI began its observations. Baltay’s team at Yale played a critical role in this effort by rebuilding and installing the Mosaic-3 Infrared CCD Imager on the Kitt Peak telescope, allowing for enhanced mapping of critical targeting data. But finding these galaxies was just the first step. Next, another Yale team, headed by research scientist and imaging expert David Rabinowitz, developed the Yale FiberView Camera. The device enables the efficiency of the DESI system by guiding the robotic positioners, which ensure each of its five thousand optical fibers are aligned on its preselected target galaxy. “It’s measuring spectra for five thousand galaxies in a single exposure […] and they have to have a particular spectrum that you know well enough in advance, so that when it’s shifted because of its distance, you know,” Rabinowitz said. Through this work, the Yale team’s contributions have played a crucial role in the success of the DESI project, which has now collected data on over thirty million galaxies.
In its first year of data collection, DESI has already constructed the largest and most precise 3D map of the universe, offering a glimpse into whether our current understanding of the cosmos—the Lambda CDM model—still holds true. DESI’s current data supports the Lambda CDM model and the predictions of general relativity at cosmic scales. Yet, DESI’s early findings also hint at the fascinating possibility that dark energy may not be a constant force, but evolving over time. Therefore, DESI’s current results still cannot rule out alternative theories of modified gravity—hypotheses which suggest general relativity may not fully apply to vast cosmic scales. Other important findings of DESI’s measurements have included further contributions to scientists’ calculations of the Hubble constant and setting new upper limits on the mass of neutrinos, the only fundamental particles whose precise mass remains unknown.
Expanding Horizons
As DESI continues its five-year mission, each new data point will bring us closer to answering these fundamental questions. Will the Lambda CDM model prevail, or will new perspectives on dark energy’s evolution spur an entirely new cosmological understanding? DESI’s journey is only the beginning; plans for DESI-II, an extension set for 2029–2035, aim to continue this research, while next-generation projects like the Stage-5 Spectroscopic Experiment promise to map multiple times as many galaxies as DESI.