Photography by Paul-Alexander Lejas
You may not feel it, but the Earth is moving underneath your feet. Plate tectonics—the processes that move the rigid, cohesive, rocky plates of Earth’s crust—is something most of us take for granted, despite its profound effects on the state of the planet. How long plate tectonics have been operating on Earth has long been a subject of debate, with implications for our understanding of the evolution of the Earth’s early climate, heat budget, and habitability.
A recent study published in Nature Communications, co-authored by Yale researchers, provides new evidence that suggests Earth’s plate movements may go back as far as 2.5 billion years to the Neoarchean Era—pushing the onset of tectonic activity further back than ever before.
A Lot on Our Plate
The question of when plate tectonics first began is far from simple. Some researchers argue that modern plate tectonics goes back around seven hundred million years, while others contend it emerged nearly three billion years ago. This broad range exists in part because modern plate tectonics involves a suite of coupled processes beyond just the movement of plates. Theories of plate tectonics incorporate volcanic activity, mountain building, and rock recycling via plate subduction and ocean ridge spreading. While geologists can find evidence of one or more of these processes operating in the distant past, that does not mean the whole system functioned the way it does today.
Instead, researchers must look for clues as to what processes were taking place at each time, evaluating each of the criteria by their own separate evidence. “There [are] many ways to do that, but one of them involves what my lab does: the magnetism of rocks, which we call paleomagnetism,” said David Evans, professor of Earth and planetary sciences at Yale and a senior author on the study. While other methods may interrogate the rock record for evidence of volcanic activity or remnants of plate subduction, paleomagnetism looks at the big picture: rocks moving around the world in the processes sometimes referred to as plate mobilism.
Paleomagnetic analysis leverages the fact that crystalline minerals in some rocks act like tiny bar magnets, recording a snapshot of the Earth’s magnetic field at the moment the rock was formed. The analysis involves carefully measuring the magnetic orientations of rock samples containing these crystals, with the knowledge that the rocks’ orientation may have been tainted by intervening magnetic processes. “You could have a magnetic mineral that has the right size and chemistry to hold a magnetic signal for billions of years, and it still might be secondary,” Evans said. With some trigonometry and good accounting for any post-crystallization changes in magnetic orientation, such as remagnetization by later heating events or physical deformation, geologists can estimate how far the rock was from Earth’s magnetic poles when it was formed. By carrying this analysis out for many rocks of different ages, geologists can reconstruct the motions of any tectonic plate over long periods of time.
The smoking gun that geologists look for in support of early plate mobilism is independent movements of ancient crustal blocks. These blocks are called cratons, which preserve some of the oldest rocks on Earth and typically make up the “cores” of modern continents. “One of what we think are the necessary requirements for plate tectonics is that you’ll have substantial motion across the Earth’s surface—not just motion of one piece of real estate across the surface, but two pieces that are moving independently of each other,” Evans said.
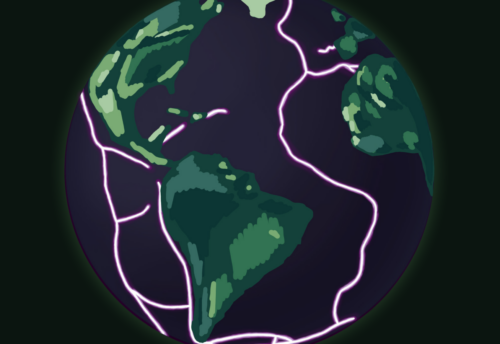
Previous paleomagnetic studies have shown the apparent motion of one craton up to three and a half billion years ago, but that data might also be explained by a phenomenon called “true polar wander.” True polar wander occurs when the entire planet shifts relative to its spin axis, settling into a new rotational equilibrium. This global rebalancing usually results from slow deformation of rocks within the Earth’s interior, changing how mass is distributed. If more mass shifts toward the planet’s axis, Earth rotates slightly faster, whereas if mass moves outward, the rotation slows down. These changes can subtly alter the length of a day as well as the orientation of the planet’s rotation. Because true polar wander involves shifting the entire planet relative to its spin axis, it can produce effects that resemble the shifts observed in tectonic plates within paleomagnetic records. If geologists obtain data from two cratons from the same period, they can check whether the plates are truly moving relative to one another or merely appearing to move because of the true polar wander phenomenon.
A Rift Between Geologists
Scientists have debated for decades the timing of the first movements of the Earth’s crust that resemble modern plate tectonics. The Neoarchean period, which spans from 2.8 to 2.5 billion years ago, has been central to this debate, as some models suggest that early Earth’s crust was largely immobile during this time. In these models, the idea goes that heat from deep within the Earth generated slow-moving currents that circulated below the crust, somewhat like the firm layer of cheese atop a bowl of hot French onion soup. This would have kept the crust’s plates from breaking up and moving around.
However, this study provides compelling evidence to the contrary. “We think the Neoarchean was not a stagnant lid because our study illustrates that the plates were moving,” said Jikai Ding, a former postdoctoral researcher in the Evans Lab and first author of the study. This movement suggests that continents were already shifting in a way reminiscent of modern plate tectonics, indicating that large-scale mobility of Earth’s crust and mantle began much earlier than previously confirmed. By establishing a new minimum age for this process, the study provides a crucial reference point for refining models of early Earth dynamics. “We found strong evidence of relative horizontal motion between Wyoming and Superior cratons before 2.5 billion years ago, which is critical for understanding when and how Earth’s early continents started behaving more like they do today,” Ding said.
Today, the Wyoming and Superior cratons make up much of the heart of the North American continent. Based on paleomagnetic data, however, we know they were separated by a wide ocean 2.7 billion years ago. So, we know they were once distant from one another, and we know they eventually collided together to move as one block. Relative plate motion must have occurred in between these states.
Elucidating the intermediate steps was where Ding, Evans, and their colleagues stepped in. By analyzing the paleomagnetic orientations of rock samples from multiple generations of mafic dikes—long, narrow igneous formations that record ancient magnetic orientations—the researchers built a robust case for independent plate motion from 2.7 to 2.5 billion years ago. The study incorporated additional geological evidence, such as the timing of craton assembly and correlations in igneous activity between Wyoming and Superior, strengthening the argument that large-scale mobility was occurring.
However, while the study supports ancient plate mobility, it does not necessarily confirm that the full plate tectonic cycle as we see it today was taking place billions of years ago. A defining feature of modern plate tectonics is subduction—where one plate dives beneath another into the mantle, recycling crustal material. No definitive geological evidence of this process was found from the Neoarchean period. “That’s why we call it a ‘mobile lid’ rather than full plate tectonics,” Ding explained. “Although calling it plate tectonics might have been more exciting and well-received, we have to respect the evidence. Science requires precision.”
While the study stops short of confirming modern-style plate tectonics in the Neoarchean, it provides the clearest evidence yet that Earth’s crust was already mobile by this time. This discovery has far-reaching implications for our understanding of Earth’s thermal evolution, the formation of early continents, and even the origins of life, which may have been influenced by tectonically driven chemical cycling. By refining our knowledge of when and how Earth’s dynamic crust began shifting, researchers are uncovering crucial insights into the planet’s earliest history.
Shifting Towards the Future
As with any breakthrough, this study raises new questions. Researchers are now looking to other ancient cratons across different continents to see if similar patterns of motion can be detected. “Our work adds to the growing debate on when modern plate tectonics began. The next step is to gather more data from regions like India, Australia, and South Africa to test whether other ancient cratons also show signs of early plate motion,” Ding said.
Future work will involve refining paleomagnetic techniques to extract more reliable data from ancient rocks, as well as integrating geochemical and geodynamic modeling approaches to better understand how early Earth operated. The ultimate goal is to determine when full plate tectonics—including subduction—became a dominant process on the planet.
As researchers continue to investigate, one thing is clear: Earth’s early history was more dynamic than we once thought, and the story of our planet’s shifting crust is still being written.