A single drop of seawater contains nearly ten million viruses.
But despite this strength in numbers, marine viruses have failed for decades to win attention. Scientists believe that only one percent of all the species of saltwater viruses have been identified to this day.
Recent discoveries in this branch of microbiology are finally shedding light on just how intriguing and powerful these viruses can be. In fact, there are ten times as many saltwater viruses as there are all other saltwater microbes combined, and their sheer number makes them an incredibly powerful ecological force. By controlling bacterial populations, marine viruses determine how much energy is available for plants and animals in the world’s oceans. They can also benefit research in agriculture, medicine, and evolution.

Image courtesy of Frederick Murphy.
One significant breakthrough in the field came in February, when Nature published a study led by Oregon State University microbiologist Stephen Giovannoni. Giovannoni’s team identified a new species of marine virus that attacks SAR11 bacteria, the most abundant marine bacteria worldwide. This discovery might not come across as astonishing. After all, 99 percent of marine viruses are still floating around unidentified; ecologists who sift through saltwater are likely to find something new eventually. And yet these new viruses, which Giovannoni has found are the most abundant saltwater viruses in the world, managed to escape discovery for decades.
The new viruses, dubbed “pelagiphages,” play a critical role in the ecology of marine ecosystems, making it even more remarkable that they remained hidden from the scientific community for so long. By regularly attacking and killing SAR11, the pelagiphages prevent the bacterial population from overrunning an entire ocean ecosystem. Paul Turner, a professor of ecology and evolutionary biology at Yale, explained that bacteria like SAR11 are extremely efficient at using their resources. Without marine viruses to kill as much as 50 percent of saltwater bacteria every day, rampant bacterial growth would quickly deplete the ocean’s resources.
SAR11 also happens to be a major player in the carbon cycle. The bacteria absorb organic carbon from the environment in order to produce energy, releasing carbon dioxide in the process. Although each individual bacterium consumes a small amount of carbon, the entire population uses up a tremendous amount of the organic carbon molecules dissolved in seawater. Given carbon’s essential role as a biological building block — it is a component of proteins, lipids, carbohydrates and nucleic acids — it is crucial that plants and animals have access to organic carbon from the environment. When pelagiphages penetrate and kill the bacteria, this captured organic carbon becomes available to other plants and animals in the water.

The inner workings of the pelagiphage resemble those of most other viruses. Technically, the virus is not alive — instead, it relies on a host cell to function and reproduce. It attacks its host, SAR11, by penetrating the bacterial cell and inserting its own viral DNA. Once it has control of the cell, it uses the cell’s machinery to make copies of itself. The SAR11 host cell eventually bursts and dies, releasing new copies of virus that are free to scatter and infect new hosts. More importantly, when SAR11 bursts it releases carbon, among other essential nutrients, into the environment.
In addition to uncovering the new virus’s role in the ecosystem, Giovannoni’s research highlights an ongoing evolutionary arms race between SAR11 and marine viruses. Because of their fast life cycles and quick reproduction, both microbes are able to evolve rapidly, and according to Turner, they are the “champions of adaptive change.” With each generation, the viruses are responding to SAR11’s evolutionary advantages with changes of their own; meanwhile, SAR11 bacteria quickly adapt to any adjustments made by the viruses. This evolutionary process is extraordinarily rapid. Viruses proceed through an average of 1.2 generations per day. By contrast, observing evolution in humans is impossible because it takes decades for multiple generations to elapse.
The arms race between SAR11 and its viral predator has been used by scientists to explain the great diversity of marine microbes. For instance, as SAR11 adapts to the virus, perhaps by developing a less penetrable nucleus or establishing a counterattack, the single strain could potentially diverge into multiple species.
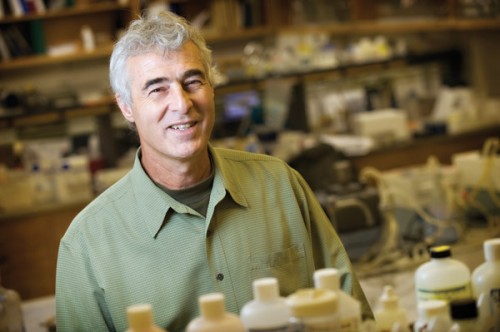
Luckily, for each new bacterial strain that emerges, there are dozens of viruses that have yet to be revealed. In July, researchers at the University of Arizona identified twelve new types of bacteria-killing saltwater viruses. “Studies like this convince us that we only know the tip of the iceberg when it comes to the diversity of viruses in natural environments,” said Turner.
Several months earlier, researchers at Cornell University added to the growing list of discoveries in marine virology. The team identified a species of saltwater virus that attacks crustaceans called copepods. These crustaceans feed on photosynthetic phytoplankton, and the pellets they release sink to the ocean floor. But these pellets are not entirely waste — they include large amounts of locked-up organic carbon that came from the phytoplankton. Once again, marine viruses come to the rescue by controlling copepod populations and maintaining suitable levels of organic carbon in the ecosystem.
Given the great variety of marine viruses, cataloging these species is a tremendous step in revealing the diversity within underwater ecosystems. “One of the ultimate goals for ecologists is to eventually describe all the creatures on the planet,” said Turner. With such vast quantities of marine viruses, it is impossible to pursue this goal without exploring and identifying pelagiphages.
Of course, most people outside the field of ecology are not quite so passionate about cataloging every creature on earth, understanding the carbon cycle, or testing evolutionary theories at high speed. However, these newly discovered marine viruses have a profound impact on other aspects of research. For example, studying new viral specimens gives scientists a better understanding of how viruses can easily penetrate a host cell and navigate a victim’s immune system. By studying this process, researchers can potentially develop better anti-viral treatments to human and animal diseases.
Turner explained that other advantages of marine viruses are less obvious, but just as exciting. “We tend to only focus on viruses that make us sick,” he said, “but, if we look at specific viruses and at the specific genes that make those viruses successful, we can take advantage of viral functions for our own applications.” According to Turner, recent research has shown that certain plants can grow more efficiently at high temperatures when infected by a virus. This ability can be harnessed to support plant growth even as global warming threatens agriculture.
As research in marine viruses accelerates, Turner said he is optimistic about where the field will go. There is still much work to be done in cataloging the diversity of these saltwater viruses, and along the way scientists can continue investigating the impact viruses can have on medicine, agriculture and biodiversity. So much remains to be explored in that single drop of seawater containing ten million viruses.