What if the building blocks of life could be used to create better computers? For scientists at Stanford, that goal is one step closer to becoming reality.
Professor Zhenan Bao and her team are finding ways to assemble computer chips out of graphene, a two-dimensional molecule of linked carbon atoms with the potential to transform the electronics industry. She and her colleagues may have found the magic ingredient necessary for quickly and automatically assembling graphene into the transistors found in computer chips.
According to the paper published in Nature Communications, DNA, the instructions our bodies use to build cells, is a long and skinny molecule with the perfect structure for building transistors. Bao’s team developed a way to use DNA as a template, assembling graphene ribbons that follow the shape of the DNA chains. The resulting graphene transistors, which can be integrated into computer chips, last longer and are more compact than their silicon counterparts.
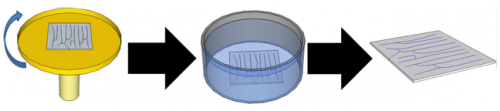
Transistors conduct electricity and have the unique ability to turn their electrical currents on and off. The computer understands this as binary code, a series of ones and zeros that store data. Bao’s team worked to build field-effect transistors, which are the most common type used in industry today. These are built from long, thin strips of semiconducting material that can easily disrupt the flow of current.
Transistors run in parallel to their neighbors, so the more transistors a computer has, the faster it can operate. The computing industry is perpetually trying to improve its operating speed, but the limitations on transistor size have hindered this race.
So far, silicon has been the favorite semiconductor for building transistors. It is an extremely powerful element that is responsible for the impressive computing power of modern machines. However, it does have limits. Silicon transistors cannot be built below a critical size or else they will break down. In addition, the insulating bands of semiconductors wear away over time, leading to current leakage.
Graphene, a single sheet of carbon only one atom thick, has the potential to transform the transistor industry. It has been a popular subject of study ever since its discovery in 2004 by Andre Geim and Konstantin Novoselov. The two scientists won the 2010 Nobel Prize in Physics for their ingenious method of producing graphene from graphite, a common related compound. Graphite is made up of layers of carbon sheets that slide against each other; using Scotch tape, Geim and Novoselov removed the layers one by one until they had produced a single isolated sheet — graphene. Scientists have found that when a sheet of linked carbon is separated from its neighbor sheets, it gains some remarkable properties: Graphene is an excellent conductor, very light, and extremely strong. It is also in a very stable configuration.
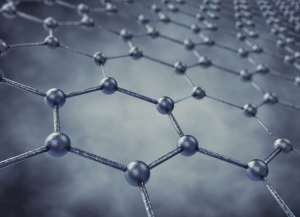
Carbon sits directly above silicon on the periodic table. Both elements have semiconducting properties, but carbon is able to better withstand heat and degrading environments. Up until now though, silicon in its pure compound form has been vastly easier to manufacture than carbon semiconductors. And while the Nobel Prize-winning method of producing graphene is reliable for creating sheets, it does not have great control over the shape or dimensions of the sheets. Producing transistors requires long, thin ribbons of graphene.
Bao’s lab at Stanford is attempting to create a system for mass producing these ribbons by using DNA from bacteria. By using the DNA as scaffolding for assembling the graphene, the researchers found that they could grow the graphene into the desired shape.
The first step in this process was chemically straightening the DNA through a process known as “combing.” Combing pins the ionized, or “frayed,” edges of a strand of DNA to a surface of liquid. The DNA stretches taut as the liquid evaporates, just as skin feels tight in the winter after all the moisture has been sucked out of it. Next the DNA is bathed in copper salts, which are quickly absorbed into the chains. The salts break up some of the bonds within the DNA strand, leaving strings of carbon ions eager to bond with any compound they can latch onto.
After the salts have been fully absorbed into the DNA, methane, a carbon-rich gas, floods the surface above the DNA. The system is heated up to 1000° C, providing enough energy for the exposed carbon strands in the DNA to readily bond to the carbon in the methane. The other elements of the DNA evaporate in the heat, and the carbon compound deposits onto the surface below the DNA, effectively creating a shadow projection of the chains. These projections are the long, thin ribbons of graphene that the researchers were hoping to manufacture.
To confirm their experiments, Bao’s team built a working field effect transistor from their graphene strips, successfully moving current across the device. They also discovered that strips that had been created without the help of copper ions were nonconducting, thus reaffirming the need for copper salts in the deposition process.

To demonstrate the potential of the DNA templating process, Bao’s team also modified the initial “combing” procedure, creating a crossed network of DNA instead of a linear one; as expected, carbon was deposited on top in the exact same shape. In her paper, Bao suggested that the designs of the graphene would be only limited by the creativity of the DNA comber. Future projects could even involve “DNA origami,” the twisting and shaping of DNA into interesting geometric scaffolds.
In Stanford Engineering’s press release, Ali Jarvey, an industry expert, praised the method. “This technique is very unique and takes advantage of the use of DNA as an effective template for controlled growth of electronic materials,” he said. “In this regard the project addresses an important research need for the field.”
The DNA method is mechanizable, so these ribbons have the potential to be produced in a factory in the near future. The DNA strips could be combed by the millions, and the graphene strips would be farmed en masse.
However, Bao admits in her paper that the method needs to be perfected before it becomes ready for large-scale manufacturing. Many of the strips deposited in multiple layers are more than an atom thick. These strips are graphitic, not graphene, and lack the necessary conducting abilities. Before mass production can start, the team needs to find a way to only allow for one sheet of deposition per strip.
Nevertheless, Bao’s discovery has made graphene a powerful new player in the transistor industry. While household graphene transistors are still several years out, there is no doubt that this exciting new technology has vaulted some major hurdles of classical computing.
Cover Image: DNA provides the template for assembling graphene ribbons, and heat kick starts the chemical reaction that creates the strips. Courtesy of Anatoliy Sokolov.