As evening falls at Mason Laboratory, after students have shuffled out and most labs have locked up for the night, a single fly takes off. Buzzing around erratically, almost confusedly, it looks lost at first. Soon, though, it is joined by another fly, and then a third. As more and more take flight, something strange happens. With seven or eight flies in the air, the scene is still chaotic—a loose-knit flurry of individual flies. But as a few more join—a ninth, tenth, and eleventh—the turmoil transforms. The ball of flies becomes uniform, and individuals get lost in the whirling, bustling group. These flies have formed a swarm.
The Power of Ten
The nature of this transformation is the subject of recent research by Nicholas Ouellette, Associate Professor of Mechanical Engineering at Yale. Ouellette’s lab studies the science of swarms—or, more generally, the dynamics of collective animal behavior. Using a colony of midges, a type of small fly, as a model system, Ouellette and his colleagues are trying to figure out questions like how many individuals it takes to make a swarm. Their surprising answer was published last month in the Journal of the Royal Society Interface: just ten. And this is only one of many surprising and enlightening results emerging from the nascent science of swarms.
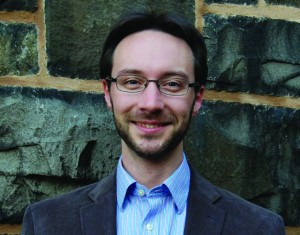
But what exactly is a swarm? “That is a much deeper question than you might expect,” says Ouellette. While most of us have an intuitive notion of what it means to swarm—think bees—defining the notion precisely is much trickier. Ouellette distinguishes between swarms and other animal groupings like flocks or schools, which tend to move as units. “A swarm is a collective behavior that doesn’t show net ordering,” he says. But Ouellette acknowledges that many other researchers would disagree. In fact, defining “swarm” fruitfully is an active area of Ouellette’s research, and a focus for other swarm scientists as well.
Unanswered Questions
The appeal of swarming for scientists is the rise of complex patterns and behavior from the collective action of many individuals. This phenomenon, known as emergence, is a key feature of swarms. For Ouellette and other researchers, connecting the emergent properties with the underlying individual behaviors is a consuming problem. “What kind of local interactions do you need to make a swarm?” Ouellette asks in his research. “And how would you model that?”
With the right local interactions and enough individuals, a swarm results. At this point, the collection of animals exhibits macroscopic properties that truly belong to the group as a whole, rather than its members. Ouellette makes the analogy to thermodynamics, where thinking in terms of macroscopic properties is more familiar. Temperature and pressure are natural examples. Similar measures can be used to characterize swarms, except the individuals are flies, bees, or birds rather than molecules.
But this is a very big difference, and perhaps the most interesting research questions focus on these contrasts between swarms and chemical or physical systems. While chemists can accurately think of their particles as bouncing about at random, swarm scientists cannot. Midges fly under their own power; they are active agents rather than passive particles. And while the large-scale behaviors of chemical aggregates are generally well understood, animal swarms remain more mysterious. Ouellette uses empirical approaches, like filming and tracking the midges, to shed light on how they form and behave. His lab has worked to evaluate which models of swarming match up best with biological reality.
They also ask questions like the one about the size of swarms. To determine the minimum number of midges in a swarm, Ouellette and postdoctoral researcher James Puckett, now an Assistant Professor of Physics at Gettysburg College, recorded many swarms of midges of various sizes. They measured characteristic properties of these swarms, including the average distance from one midge to another and the average velocity of each midge. By plotting each property against swarm size, they could better understand how a group of midges becomes a swarm. They found that every property they examined leveled off rapidly, having a consistent value for large swarms of any size. Once the number of midges reaches a sort of critical mass, a swarm forms, and its characteristics remain similar no matter how many more flies are added. Somewhat surprisingly, in each case this leveling off occurred for swarms of less than ten individuals. Thus, they conclude, it only takes ten midges to behave like a swarm of many more.
The surprises, uncertainties, and hazy definitions all reflect the novelty of swarm science. Although humans have observed and interacted with herds, flocks, and swarms for millennia, only recently have these phenomena—and the differences between them—become accessible to scientists. Ouellette believes that tools for studying swarms have allowed the field to grow rapidly in recent years. These tools include inexpensive and easily accessible digital imaging technologies for recording swarming behaviors, and computer vision programs that allow researchers to track individuals within a swarm.
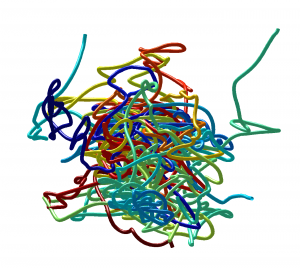
The Appeal of Swarms
Ouellette himself became interested in swarming after developing computer vision tools for the study of fluid mechanics. Following particles in a swirling pool isn’t so different from tracking midges flying in a cloud. The parallels made it easy to dip into biology. His progression isn’t uncommon; Ouellette says that thanks to technological advances, “physicists and engineers have a lot of opportunities to look at classically biological problems.” The mix of backgrounds among swarm scientists and the fertile intellectual ground they are exploring make for a heady combination. The explosion of interest among researchers is mirrored by the popularity of books like The Perfect Swarm by Len Fisher or Peter Miller’s The Smart Swarm. “Any time you have a problem with a real interdisciplinary focus, scientists get excited,” Ouellette says.
Studying swarms isn’t only an intellectual exercise, though. While the field is still very young and focused on exploring basic concepts, according to Ouellette, the applications for its findings are already exciting interest. The relevance of swarms for robotics is possibly most clear. Borrowing from the behavior of such simple animals as midges, engineers could build artificial swarms that exhibit complex behaviors without complex programming. Indeed, a number of researchers have already made forays into robot swarms. A team of Hungarian engineers, for example, recently conquered the challenge of building drones that can form a self-organized swarm in outdoor conditions. Ouellette says that autonomous robotic systems like this are the first real applications of swarm science.
It isn’t hard to imagine how robot swarms could be put to use. Imagine small robo-coptors patrolling disaster areas, looking for survivors, or agricultural robots swarming through fields harvesting crops. It may be telling that Ouellette’s own research is supported by a grant from the U.S. Army. Certainly, robot swarms could see deployment on the battlefield. Part of the appeal of robot swarms in such contexts is their resilience. If each individual robot contains the same simple programming, and the more complex behaviors are emergent, then individuals are expendable. As long as enough individuals (maybe ten, if Ouellette’s work applies beyond midges) remain, the swarm will not even notice the difference. Its behavior will remain the same. Ouellette says that this makes robot swarms “very fault tolerant,” a useful property for systems subjected to harsh environments or for those with very important functions.
This fault tolerance could make the lessons of swarm science even more widely applicable. Network control systems and other computer applications seem especially likely to incorporate them, Ouellette suspects. But for now, his attention is turned to the fundamentals of swarming behavior. Each evening when his midges swarm, he will turn on his cameras and computers—cutting edge tools designed to tackle the toughest problems in fluid dynamics—and hope to glean a bit more insight into their remarkable behavior. Perhaps that’s something to think about the next time you bat away a cloud of flies.
Extra Reading
- Brambilla, Manuele, Eliseo Ferrante, Mauro Birattari, and Marco Dorigo. “Swarm Robotics: A Review from the Swarm Engineering Perspective.” Swarm Intelligence 7, no. 1 (2013): 1-41.
- Kelley, Douglas H., and Nicholas T. Ouellette. “Emergent Dynamics of Laboratory Insect Swarms.” Scientific Reports 3 (2013): 1073.
About the Author: Zach Miller is a sophomore in Saybrook College studying Ecology and Evolutionary Biology and Applied Mathematics. He is currently researching the ecology and evolution of Greek lizards with Professor Oswald Schmitz and doctoral student Colin Donihue.
Acknowledgements: The author would like to thank Professor Ouellette for his willingness to give phone interviews while on sabbatical, and for the lucid explanations of his research.